Gain new perspectives for faster progress directly to your inbox.

Trends and innovations in biomedical 3D printing
We are in the middle of a 3D printing revolution. Once available to only major research universities and Fortune 500 companies, 3D printing technology has become increasingly mainstream, with 2.2 million units of 3D printers shipped in 2021 . This number is set to rise to 21.5 million by 2030, bringing this rapid prototyping technology to the masses.
Seemingly every major industry, from aerospace to construction, leverages 3D printing technology for rapid and cost-effective manufacturing. Of all industries embracing the power of 3D printing, biomedical engineering holds the greatest potential for its applications. In this article, we will explore the rise of 3D printing in healthcare and medicine.
How it all began — the history of 3D printing
When Japanese inventor Hideo Kodama filed the first patent for a “ rapid prototyping device ” in 1981, the concept seemed doomed from the start, as Dr. Kodama quickly abandoned financing the patent the following year. Yet the idea provided the catalyst for further innovations. In 1984, Charles Hall filed a patent for a stereolithography system (SLA), a 3D printing technology widely used to this day. The first commercially available 3D printer followed in 1988, based on the groundbreaking SLA technology.
Other key 3D printing technologies soon followed. By the late 1980s, patents had been filed for two further types of additive manufacturing devices: fused deposition modeling (FDM) and selective laser sintering (SLS). FDM works by a technique called extrusion, where a nozzle deposits the heated material layer-by-layer to build up the 3D product. SLS works somewhat differently; the process involves spreading layers of powder-based material over the build platform, followed by the rapid solidification (or ‘sintering’) for each layer of the 3D-printed product. Subsequently, “jetting” (a modified version of 2D inkjet printing technology) and vat photopolymerization soon followed.
These technologies were originally limited to patent holders. However, with the expiry of those patents and the invention of the RepRap open-source concept, new companies can now make a name for themselves in this exciting field. Many of the largest breakthroughs have been in the field of biomedicine, including the development of the first 3D-printed organ for transplant surgery — a bladder.
Today, 3D printing for biomedical applications is booming. The global market size of biomedical 3D printing was estimated at $1.45 billion in 2021 and is expected to rise to approximately $6.21 billion by 2030. To uncover key trends in biomedical 3D printing, we analyzed data from the CAS Content Collection™, the largest human-curated collection of published scientific knowledge.
Technologies and materials in 3D printing
3D printing falls into four broad categories — powder bed fusion, jetting, extrusion, and photopolymerization. Due to the diverse range of applications, there is no one ‘one-size-fits-all’ 3D printing technology. Extrusion-based technologies such as FDM, however, remain the most popular types of biomedical 3D printing (Figure 1).

From plastics and metals to natural substances, a vast array of materials can be utilized in biomedical 3D printing. Synthetic polymers such as polycaprolactone and poly(lactic acid) rank among the most commonly used 3D printing materials (Figure 2), owing to their applications in microfluidics and medical implants . The most widely used inorganic substance is hydroxylapatite, which is used as a dental material and a filler for bone repair. A variety of natural polymers such as alginate and hyaluronic acid are gaining popularity in bioprinting.
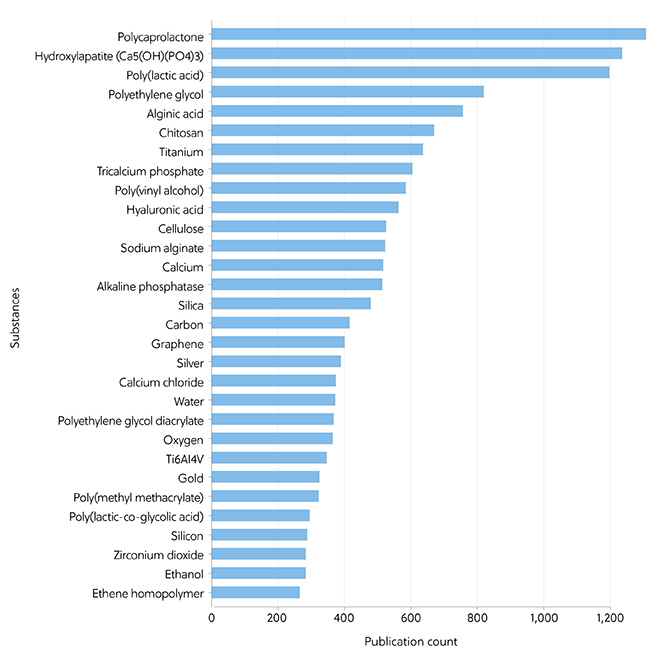
The rise of biomedical 3D printing
Annual trends of journal and patent publications for biomedical 3D printing applications indicate that innovation in this area is booming, though the number of journal publications was dramatically higher (approximately 15,000) than patent publications (approximately 5,700) (Figure 3). This trend may reflect the increased commercialization of the technology in recent years.
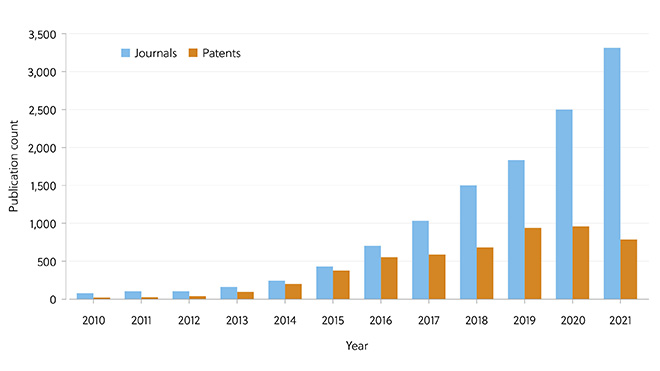
Approximately 90 countries have published papers on the biomedical 3D printing applications, hinting at a widespread interest in this technology. Of these nations, the U.S. and China led the way, having the most publications for both journal and patent publications (Figures 4 and 5).

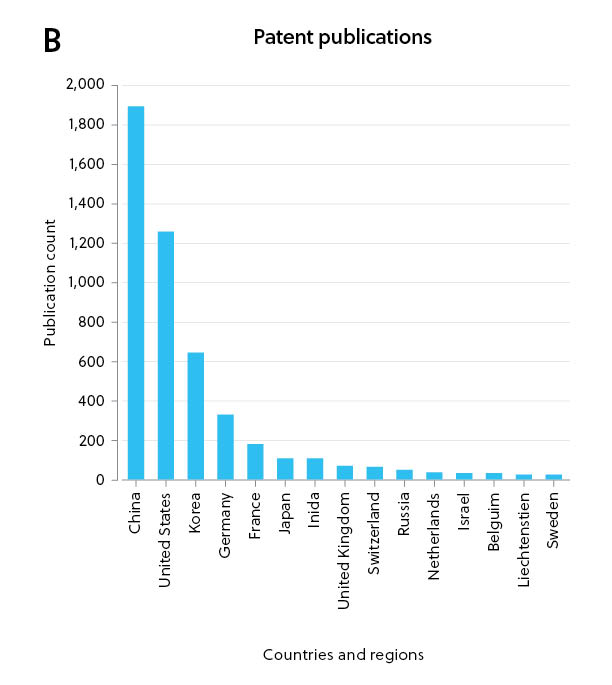
When we break down the biomedical 3D printing trend in patent assignees, we can see that most patents have been assigned to 3M, a US-based company. Other active countries in publishing patents include Korea, Lichtenstein, France, and China (Figure 6).
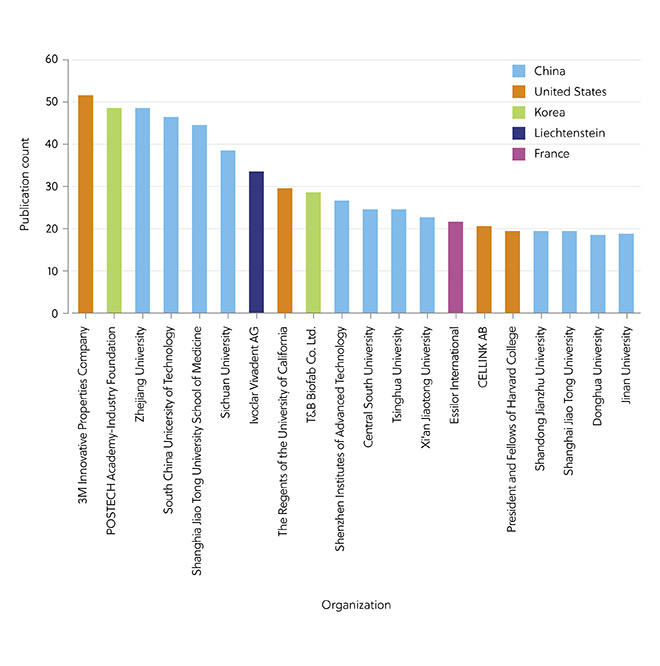
Innovative applications of biomedical 3D printing
We have already highlighted some key biomedical 3D printing applications, yet the possibilities are limitless. From the development of medical implants to the manufacture of medical equipment, innovations are coming thick and fast. Tissue and organ engineering is a major application of 3D printing, with the fabrication of complex structures such as cartilage, muscle, and skin being explored. Analysis of the CAS Content Collection shows that concepts such as “tissue engineering”, “tissue scaffolding”, and “bioprinting” appear frequently in biomedical 3D printing publications related to tissues and organs, highlighting that this is a key area of research focus (Figure 7).

3D printing technology also has several potential applications in pharmaceutics to help make the elusive goal of personalized medicine a reality. Using biomedical 3D printing, it may be possible to modify and fine-tune the dosage, shape, size, and release characteristics of pharmaceutical products.
Biomedical 3D printing technology has also opened new capabilities in creating prosthetics and implants, with the potential to create prosthetics personalized to the anatomy, color, shape, and size of the patient. Flexible materials have provided more options with body parts and capabilities, while metals like titanium alloy can be utilized in bone reconstruction . Analysis of the CAS Content Collection shows that concepts such as “prosthetic implants”, “prosthetic materials”, and “dental implants” appear frequently in 3D printing publications related to orthopedics and prosthetics (Figure 8). Though there are markedly fewer publications compared with tissues and organs, this is still a dynamic and rapidly growing field.
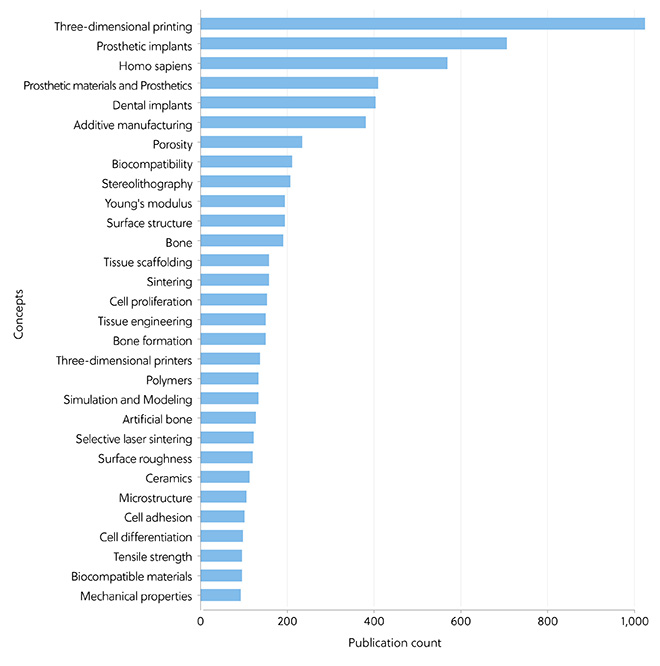
Challenges in biomedical 3D printing
Though we have seen many exciting advances in biomedical 3D printing, in many areas, the technology is still in the early stages. For instance, researchers have successfully bio-printed vascularized cardiac patches, yet fabricating a robust heart valve (let alone a full-scale organ) is still a long way from being a reality. Currently, 3D printers are simply unable to fabricate tissues with the biomechanics and functionality of the real thing. Advancements in bio-inks and the use of media and stem cells will all likely contribute to the future optimization of these methods.
The future of biomedical 3D printing
If the current research trends are anything to go by, we can expect significant continued investment and innovation in biomedical 3D printing. We predict that the technology will become more widespread, with the concept of 3D printers being used in pharmacies now becoming a near possibility. Though biomedical 3D printing represents a significant financial investment for hospitals, the benefits can far outweigh the costs with the right planning. As the technology grows, there is a need for standardized terminology and the Food and Drug Administration to define a new regulatory framework that ensures the safety and effectiveness of biomedical 3D printing products.
To learn more, download our Insight Report.