Gain new perspectives for faster progress directly to your inbox.
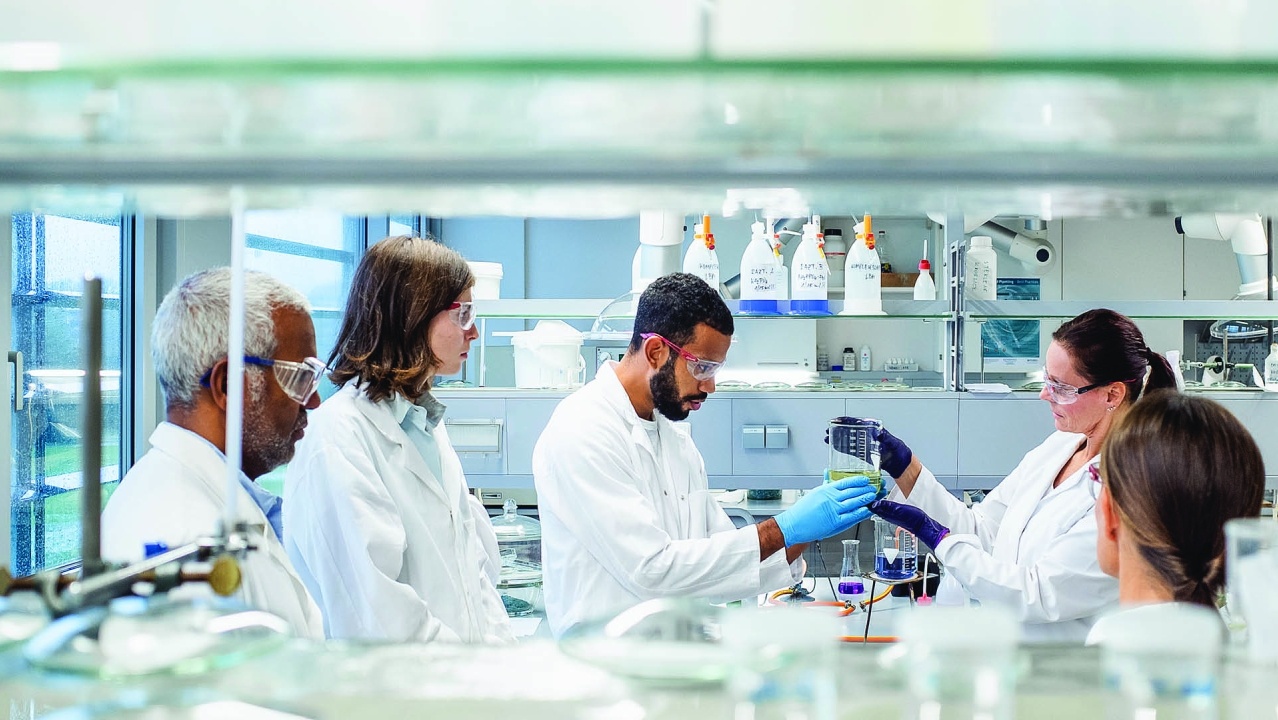
As a therapeutic class, covalent inhibitors have been gaining popularity in recent years, with the U.S. Food and Drug Administration (FDA) approving an array of covalent drugs across several indications, primarily focused on cancer therapy. Their unique mechanism of action offers several advantages over more common non-covalent inhibitors, making them a promising avenue for therapeutic development.
Historically, researchers have shied away from investigating covalent inhibitors due to safety concerns and challenges with their design and manufacture. However, the tide is turning.
CAS has identified more than 10,000 scientific articles discussing a subset of covalent inhibitors since 2000. Notably, research efforts have intensified in the last decade, with journal publications quadrupling since 2010 (Figure 1).
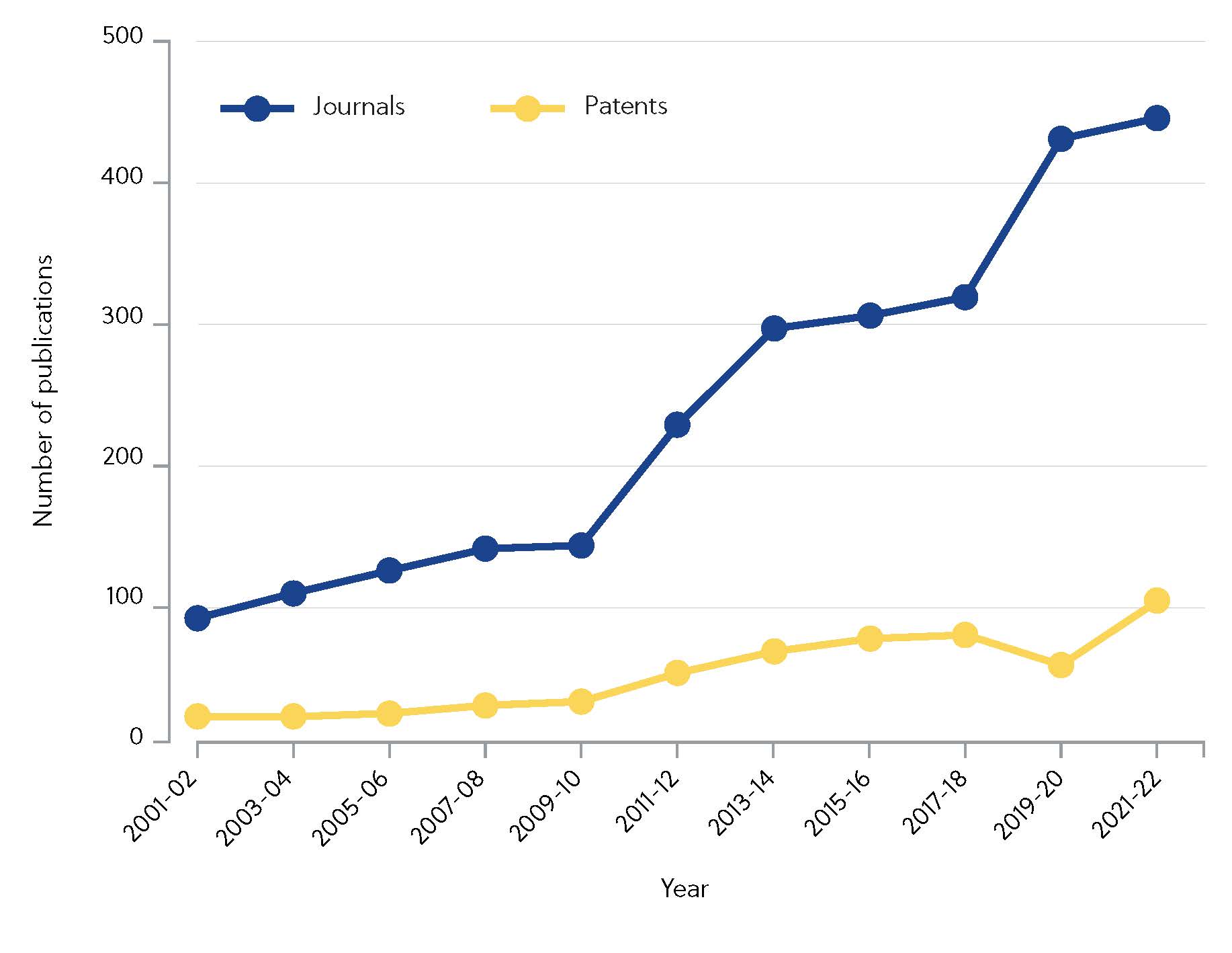
The investment is paying off, with blockbuster covalent drugs ibrutinib and osimertinib generating sales totalling $4.33 billion in 2020. The question remains: what exactly are covalent inhibitors, and why are companies and academic labs starting to favor them over non-covalent inhibitors?
As the name suggests, covalent inhibitors form a covalent bond with their target proteins, usually irreversibly modifying them. Unlike non-covalent inhibitors, which bind reversibly, covalent inhibitors create a lasting connection with their target, leading to enhanced potency and a prolonged duration of action. This can have advantages and disadvantages.
The structure of a covalent inhibitor typically consists of two parts: the reactive group or “warhead,” which forms the covalent bond with the target, and the “guidance system,” which determines the selectivity of the inhibitor for its target (Figure 2). Think of it as a heat-seeking missile: the guidance system locates the target, while the warhead “strikes” to form the covalent bond.
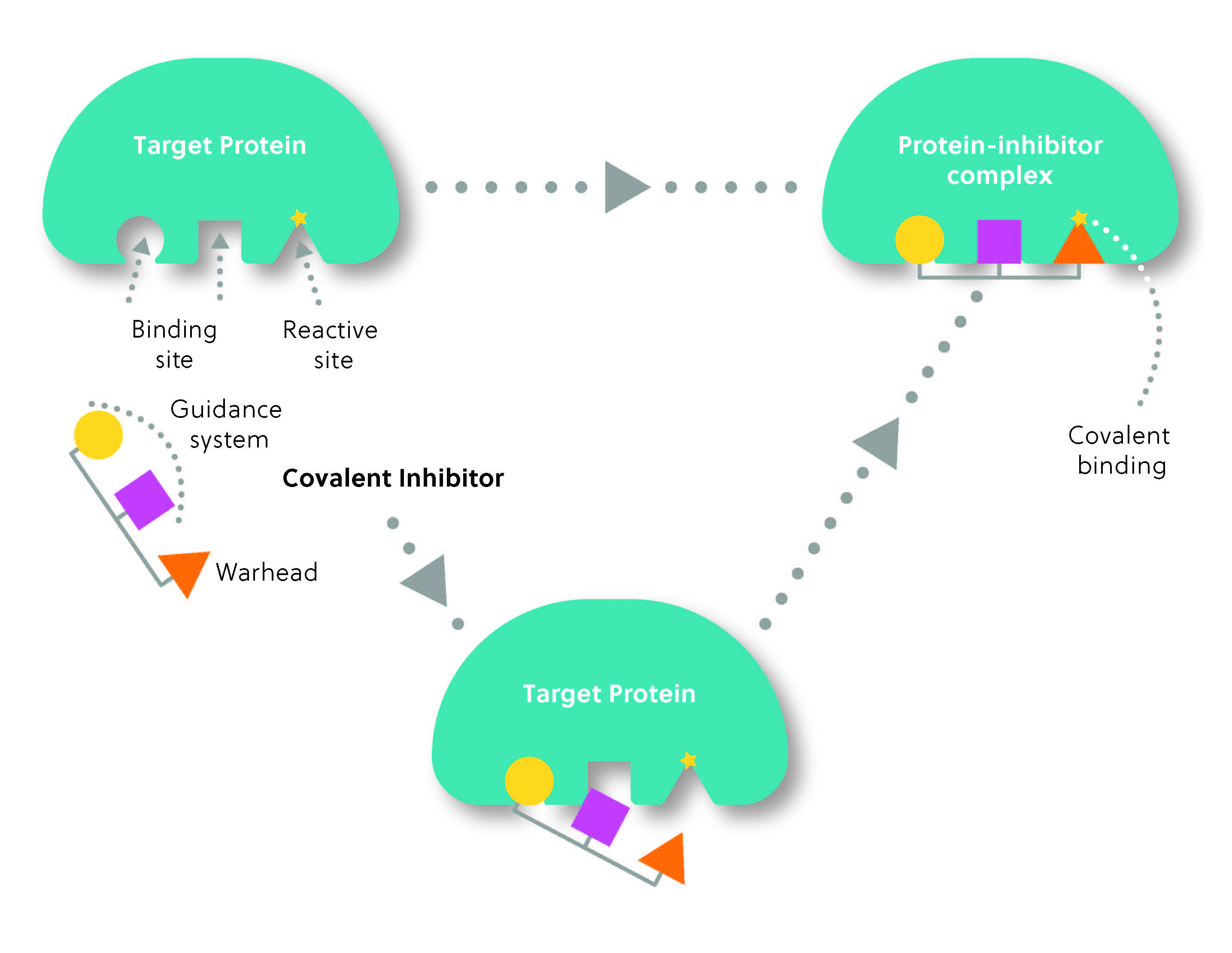
Covalent inhibitors are not a new innovation. In fact, they have been used to treat diseases for over a century, with penicillin being one of the earliest examples. Penicillin antibiotics covalently bind to their target enzyme, thereby inhibiting bacterial cell wall synthesis and leading to cell membrane rupture. Another well-known example is aspirin, which irreversibly acetylates a key enzyme involved in producing inflammatory mediators. Interestingly, the discovery that these drugs act through covalent mechanisms was made after their use was already widespread. In fact, many of the major covalent inhibitor drugs were discovered serendipitously rather than through rational drug design.
Advantages of covalent inhibitors
The potential advantages of covalent inhibitors are vast. While non-covalent inhibitors associate and dissociate freely from enzymes, covalent inhibitors form strong, lasting bonds with their targets. This means they can effectively shut down the target protein’s activity for an extended period, leading to more sustained therapeutic effects. This enhanced potency means that drugs can be delivered in lower and less frequent doses, potentially reducing the risk of side effects, increasing compliance, and decreasing treatment costs.
Additionally, covalent inhibitors can exhibit remarkable selectivity, precisely engaging their intended targets. This property enables them to target challenging proteins that are otherwise difficult for non-covalent inhibitors, such as those without conventional binding pockets. In fact, targets previously seen as ‘undruggable’, such as KRAS (the most frequently mutated oncogene), can now be successfully targeted using covalent inhibitors. The approval of the first KRAS inhibitor, sotorasib, represented a significant milestone for the management of challenging-to-treat cancers.
Disadvantages of covalent inhibitors
Many properties that make covalent inhibitors promising therapeutics can also create challenges in drug development. The irreversibility of binding requires that any binding be selective. However, if the warhead is not sufficiently selective, the drug may bind to unintended proteins, resulting in off-target effects that may lead to long-term harm. Furthermore, some target proteins may mutate or undergo structural changes that prevent the covalent inhibitor from effectively binding and inhibiting them. This can lead to the development of resistance and reduce the drug’s long-term efficacy.
Due to these challenges, designing covalent inhibitors requires careful consideration of various factors, including the warhead’s reactivity, the binding site’s specificity, and the stability of the formed covalent bond. Reactive groups must follow the ‘Goldilocks principle’ — they must be reactive enough to form a bond to a target upon complex formation but not reactive enough to form bonds to other protein targets or functional groups when unbound. Achieving the right balance between these factors can be complex and time-consuming.
The rise of covalent inhibitor therapeutics
Advancements in technology have played a crucial role in accelerating the development of covalent inhibitors. A key turning point in the field came when researchers began focusing on covalency from the outset of development rather than discovering the mechanism incidentally.
Researchers have utilized two key approaches to create effective covalent inhibitor therapies: ligand-first and electrophile-first. Ligand-first approaches involve designing small molecules that can react with specific amino acid residues in the target protein. In contrast, electrophile-first approaches focus on the warhead portion of the drug, identifying reactive electrophilic groups that can form covalent bonds with nucleophilic residues in the target protein.
To date, more than 30 covalent inhibitors worldwide have received regulatory approval as therapeutics (Figure 3), the most notable being the aforementioned ibrutinib, which hit the top 10 in worldwide drug sales in 2021. Ibrutinib is one of several notable kinase inhibitors discovered through ligand-first approaches, along with afatinib, dacomitinib, and neratinib.
Another promising application of covalent inhibitors is their ability to inhibit viral proteases. Nirmatrelvir (in combination with ritonavir), a reversible covalent SARS-CoV-2 MPRO inhibitor, has been approved for use by the FDA to treat COVID-19, providing a much-needed oral treatment option for vulnerable patients. Nirmatrelvir was developed via an electrophile-first approach through a structurally guided adaptation of a previously studied covalent inhibitor.
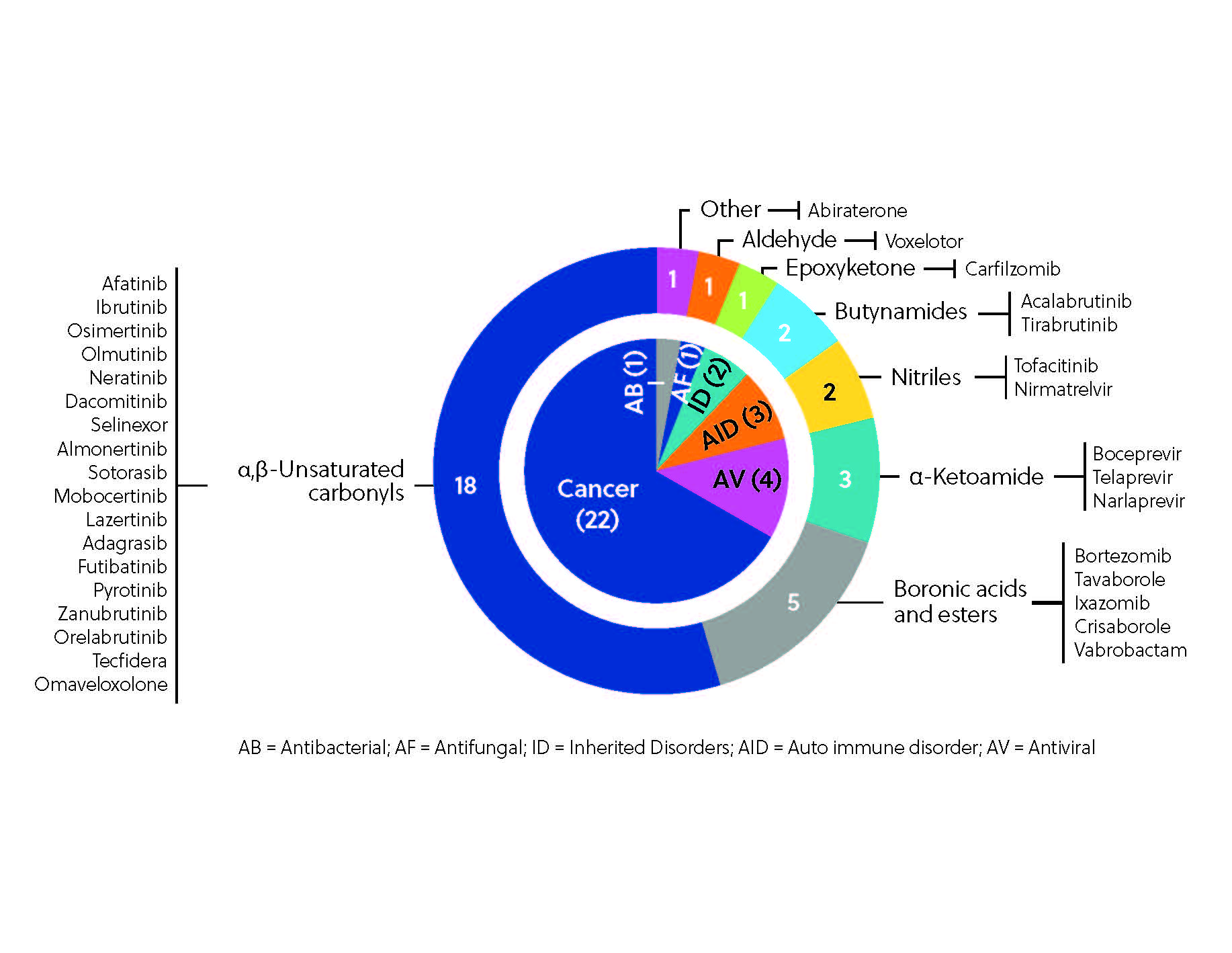
What’s next for covalent inhibitors?
The clinical development pipeline for covalent inhibitors is brimming with potential. Several covalent inhibitors are currently in clinical trials (Figure 4), including BTK inhibitor remibrutinib, third-generation EGFR-TKI nazartinib, and selective FGFR4 inhibitor roblitinib. The pipeline is diverse, with multiple proteins targeted across different therapeutic areas from autoimmune disorders such as rheumatoid arthritis and alopecia areata to other disorders such as obesity and osteoarthritis, although cancer therapies still dominate the pipeline. This variety is a testament to the sheer potential of covalent inhibition, made possible by emerging tools and techniques, including sophisticated screening platforms and chemoproteomics-enabled discovery. These technologies allow researchers to screen vast compound libraries and directly visualize covalent interactions within the proteome. We can expect even more precise and potent covalent inhibitors to emerge with these innovations.
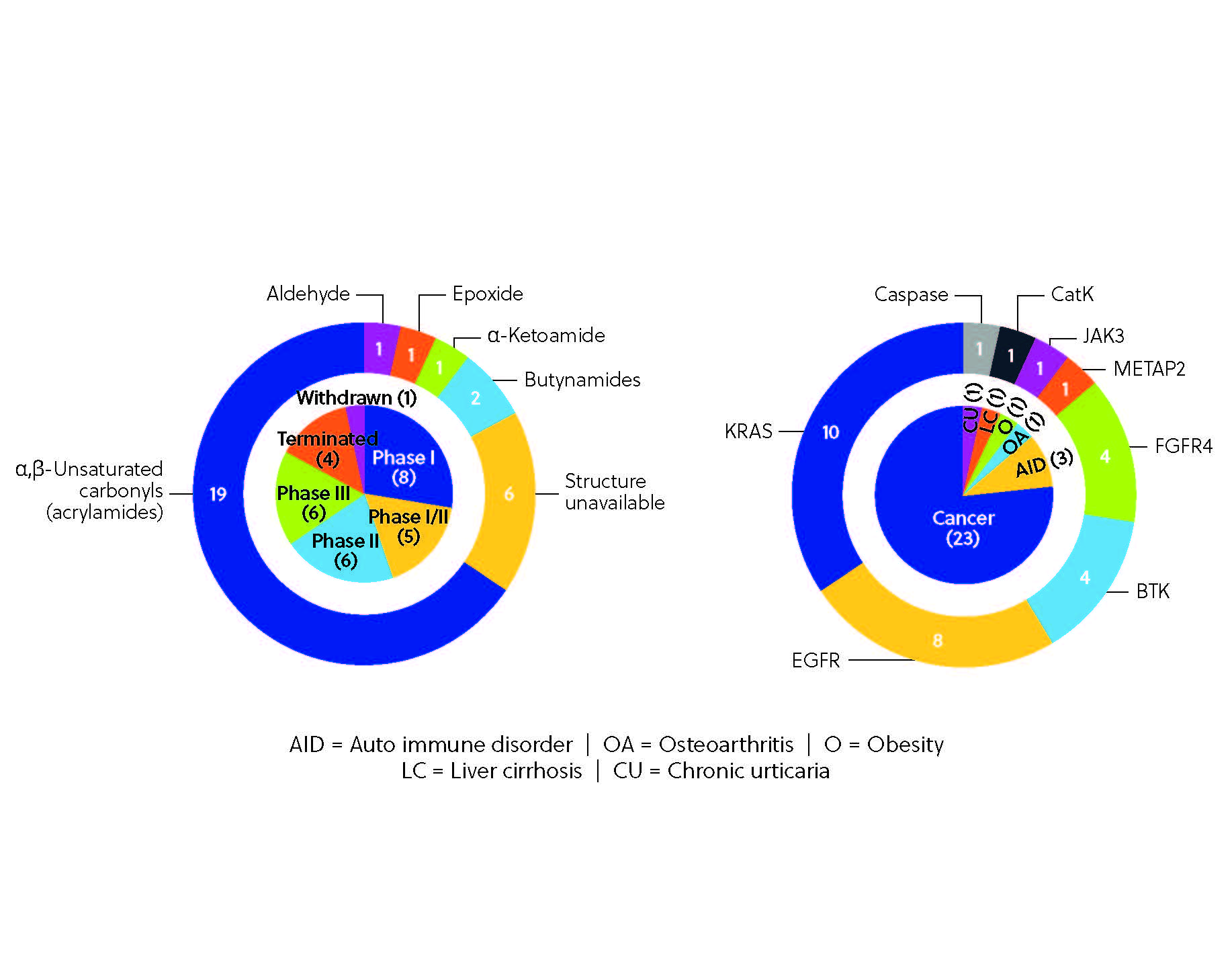
The rise of covalent inhibitors marks a turning point in drug development. Their ability to form lasting bonds with target proteins, coupled with technological advancements, has paved the way for a new era of therapeutics. As research in this field continues to flourish, covalent inhibitors hold great promise in revolutionizing the treatment of various diseases and improving patients' lives. To learn more about covalent inhibitors and hear what the experts believe are the key trends to watch, view our webinar or read more in our recent publication in ChemRXiV. For additional cancer related topics, explore our in-depth landscape analysis on RAS inhibitors or learn more about targeted protein degradation and molecular glues.