Gain new perspectives for faster progress directly to your inbox.

Supercapacitors have sometimes been heralded as replacements for lithium-ion batteries (LIBs), offering a variety of compelling advantages, including increased safety, faster charging/discharging, and longer lifetimes. Despite advancements, fundamental differences between the two technologies limit the energy density of graphene-based supercapacitor technologies, making them unlikely to replace LiBs in the future. They are, however, ready for several other real-world applications where they act as complementary energy storage devices, particularly in the transportation sector.
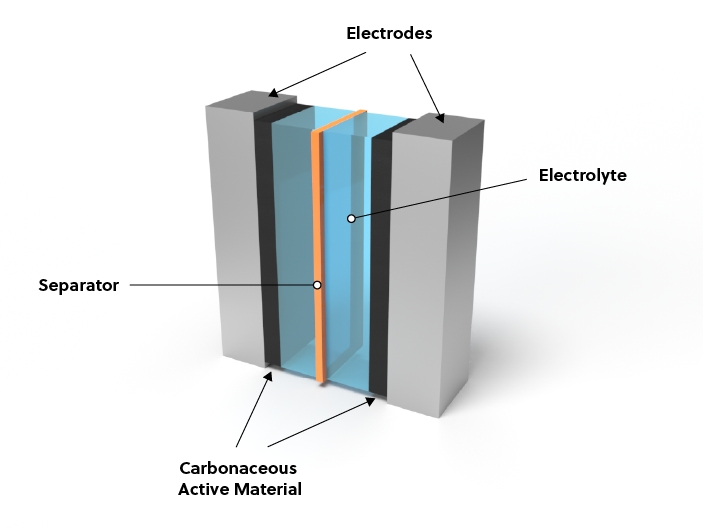
Supercapacitor technologies vs. batteries
To understand why supercapacitors have not replaced batteries, it’s important to understand the differences between these two types of devices, which stem from their architectures (Figure 1).
- Batteries have a high energy density but low power density (slower energy discharge), making them suitable for long-term applications where a consistent, slow release of energy is needed.
- Supercapacitors have a lower energy density but a higher power density (faster energy discharge). As a result, they cannot store as much energy as batteries but can be charged and discharged much faster. This property makes them more suitable for applications in which quick bursts of energy are needed and where they can be readily recharged.
The differences in energy and power density are due to the way each of these technologies stores charges, which affects their capacitance and energy density.
- Batteries store and release energy electrochemically, limiting their rate of charging-discharging by the kinetics of the corresponding electrochemical reactions. Ions are intercalated within the electrode, not on the surface, forcing ions to diffuse through the electrodes and further slowing their charging-discharging rate.
- Supercapacitors store energy electrostatically on their electrode surfaces. Energy is released by a simple movement of ions instead of a slower electrochemical reaction. Because charges (ions) are stored only on the electrode surface and no ions are intercalated within the active material, only the surface participates in the charge-discharge process, providing a much lower energy density than batteries.
Graphene is at the forefront of energy density improvements in supercapacitor technologies
Although their fundamental differences make supercapacitors unlikely to replace batteries, research is still focused on energy density improvements. Because the active material surface is the main charge storage location, research efforts are centered around developing active materials with a high surface area to increase the number of adsorbed ions, ultimately increasing the capacitance and energy density of supercapacitors.
Carbonaceous materials, particularly activated carbon and graphene, are common active materials, with graphene becoming increasingly popular due to its higher electrical conductivity. However, graphene is more difficult and expensive to produce and typically has a lower theoretical surface area than activated carbon, making it challenging to use in supercapacitors at industrial scales. Because graphene has been at the center of active material innovations in supercapacitors, it’s important to understand the various factors working against its adoption.
Key barriers limiting the use of graphene electrodes
The reliable industrial-scale synthesis of quality graphene continues to be difficult
The same properties that make graphene a great material for supercapacitor technologies require strict synthesis conditions. This makes graphene difficult to reliably synthesize, especially at industrial scales. More concerning is that, even if it can be synthesized at industrial scales, graphene may not be of sufficient quality for use in supercapacitors. One study in 2018 analyzed several dozen graphene products and found that none contained more than 50% graphene. A similar but more limited study in 2020 compared graphene and activated carbon to show that the specific capacitance of graphene-based supercapacitors was markedly lower than that of activated carbon, likely due to the presence of graphene oxide. Because these studies were performed prior to the release of ISO graphene standards in 2021, a follow-up study is needed to carefully analyze the quality of commercially available graphene.
Graphene is expensive to produce
Rigorous control over its synthesis conditions requires the use of specialized equipment and processes that aren’t ideal for industrial production, making economies of scale difficult to achieve for graphene-based supercapacitors. Once produced, graphene requires expensive, highly sensitive characterization techniques to confirm that its quality is in-line with ISO standards. This creates another significant barrier to entry, especially for smaller enterprises, and hinders the development of graphene-based supercapacitor technologies.
Graphene sheets are prone to agglomeration
Once synthesized, strong π-π interactions cause individual graphene sheets to restack and agglomerate, which reduces the electrochemically active surface area. This limits the energy density of supercapacitors. One company, Skeleton Technologies, has found a way to inhibit these interactions and prevent restacking by using curved graphene in their supercapacitors. Although news has centered around how curved graphene is a major breakthrough (a curved-graphene-based supercapacitor was reported as early as 2010), the company that sponsored this research has reported no news of further developments in almost a decade.
Academic graphene supercapacitor technology research
Recent publication trends in supercapacitor research
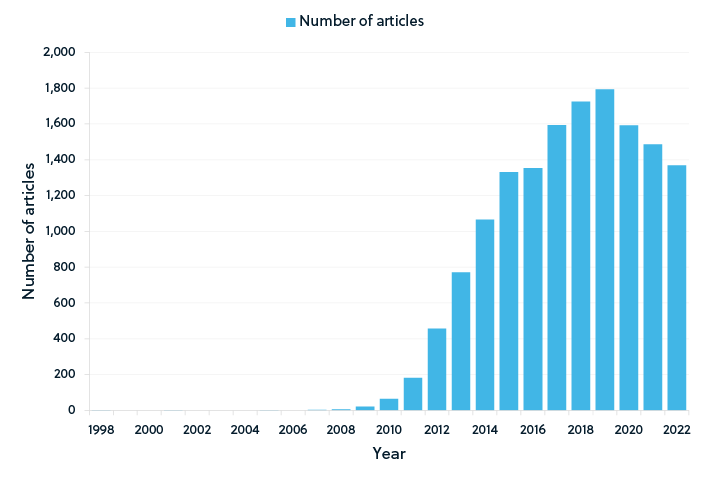
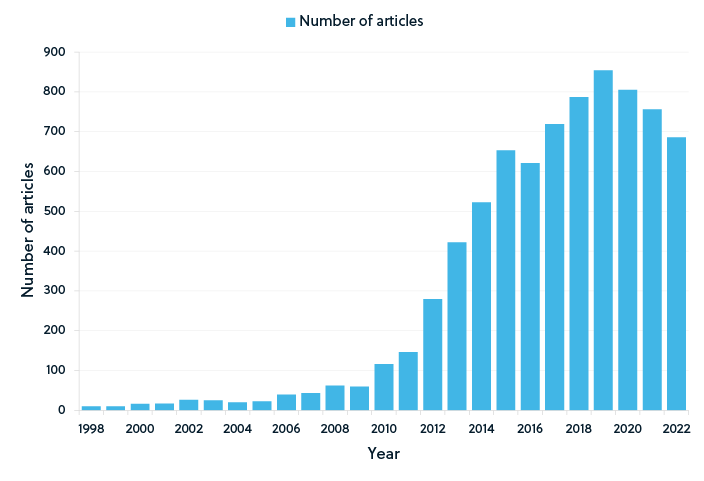
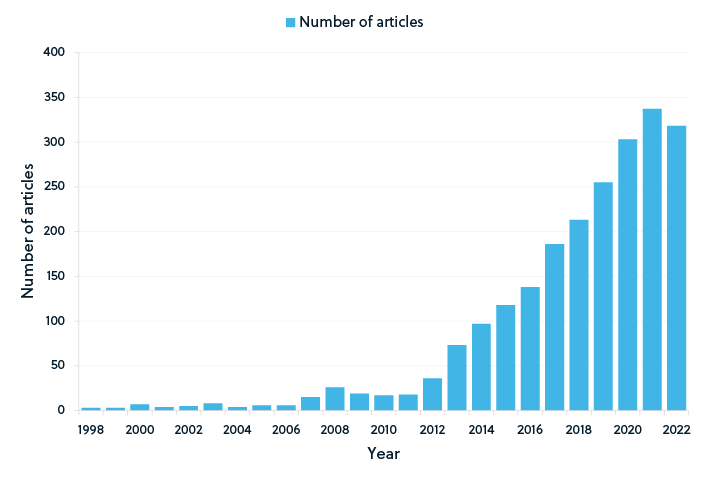
As shown by the publication trends in the figures above, graphene-based supercapacitors remain a popular research topic (Figure 2). Although graphene continues to dominate the supercapacitor research landscape, conducting polymer-based supercapacitors has also emerged as a research focus (Figure 3). While the number of publications for both graphene and conducting polymer supercapacitors began to decline slightly in 2020, publications of supercapacitors based on lignocellulosic materials continued to increase until 2021 (Figure 4), potentially suggesting an increased focus on sustainable materials.
Although academic research has made progress, the reported cycling life of lab-scale devices has often been much lower than those typically reported for commercial supercapacitors, which are usually on the order of 1 million cycles. Energy density also continues to be an issue, with the supercapacitors only able to power devices for very short periods.
- In late 2022, researchers at Tsinghua University reported a flexible graphene supercapacitor that retained almost 99% of its performance after 10,000 cycles and a charge/discharge voltage window of 3V. This supercapacitor powered several small electronic devices, including an LED and calculator, but generally for no more than a few seconds.
- In another 2022 study, a group at Imperial College London developed a knitted graphene supercapacitor. When used as a pressure sensor, it showed a rapid response time of only 0.6 seconds, but its capacitance decayed to about 90% after only 10,000 cycles.
Lithium-ion hybrid supercapacitors
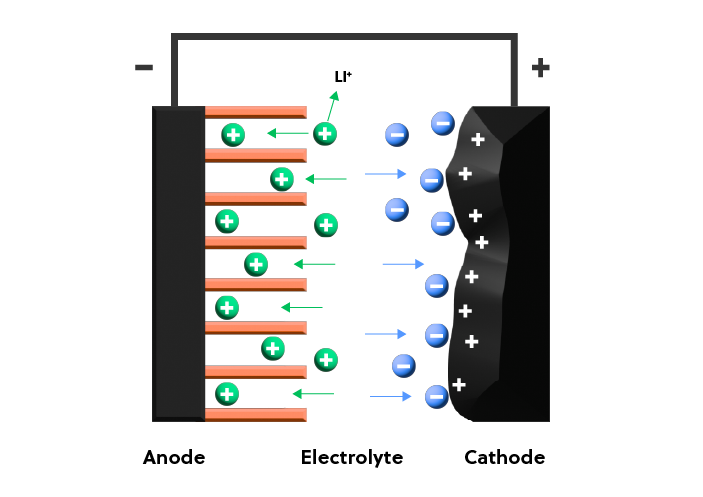
To bridge the gap between supercapacitors and batteries, different device architectures may be needed. Lithium-ion hybrid supercapacitors combine the long cycling lifetimes of supercapacitors with the high energy density of batteries. To accomplish this, the charge-discharge process involves two mechanisms: lithium-ion intercalation/deintercalation (battery-type anode) and anion adsorption/desorption (capacitor-type cathode), as shown in Figure 5. The resulting hybrid supercapacitors may show an energy density several times higher than that of a corresponding traditional supercapacitor.
However, if the capacitor-type electrode uses a graphene-based active material, it will also be susceptible to the same issues as those plaguing non-hybrid supercapacitors. Furthermore, the hybrid nature of lithium-ion hybrid supercapacitors means that while they show the advantages of both batteries and supercapacitors, they also retain some of the disadvantages. Although they may show a higher energy density and less self-discharge and leakage current than supercapacitors, they also show shorter long-term cycling lifetimes and suffer from slow lithiation/delithiation kinetics at the anode. A recent publication reported a lithium-ion hybrid capacitor that retained 100% of its capacitance after 19,000 cycles at an energy density of 100 W h kg-1.
Will supercapacitors replace batteries?
Both academia and industry are working to improve the performance of various supercapacitor technologies, but these devices are unlikely to provide long-term performance comparable to lithium-ion batteries unless fundamental limitations and engineering hurdles can be overcome.
- Supercapacitors have lower specific energy densities. Although curved graphene prevents the agglomeration of graphene sheets, supercapacitors have lower energy densities than batteries due to their different charge storage mechanisms. Without a massive breakthrough, it will continue to take several supercapacitors to rival the energy density of even a single LIB.
- Supercapacitors undergo excessive self-discharge. Supercapacitors have long cycling lifetimes and can maintain a high capacitance, but they undergo much more severe self-discharge than batteries. While a battery may lose only 5% of its stored charge over about one month, supercapacitors may lose up to 50%. This may not be an issue in applications where they can be quickly discharged and recharged, but it does affect their long-term energy storage.
- Graphene-based supercapacitors are more expensive. Because graphene-based supercapacitors are a newer technology, their production has not yet reached economies of scale. Furthermore, due to more stringent quality requirements, graphene continues to be more expensive to produce than activated carbon. While it may one day offer superior performance to activated carbon, the use of graphene also increases the prices of the resulting supercapacitors.
- Graphene-based supercapacitor applications are largely unproven. As with any new technology, the success of first-to-market products is critical to the success of subsequent product lines. None of the graphene-based supercapacitor technologies have been investigated long-term, and most have only been installed in a limited number of units.
Current and future applications of supercapacitor technologies
Transportation sector
Despite the above challenges preventing supercapacitors from replacing batteries, they have found real-world applications, but those based on graphene are still emerging. Some of the most high-profile applications have been in the transportation sector:
- Supercapacitor-powered bus fleets have been deployed in China and Serbia. One of these fleets has a reported 25 km range and takes six to seven minutes to charge. To overcome the low energy density of supercapacitors, and thus the limited range per charge, the buses are recharged either at the depot or bus stops.
- Skeleton Technologies produces a graphene-based supercapacitor for use in trains that can recover up to 30% of energy lost during braking. This technology has been selected for use in new trains for the Granada metro system in Spain, which are expected to enter service by the summer of 2024.
- Samsung advertises a lithium-ion hybrid for automotive applications that is suitable for low-voltage systems where rapid charging/discharging is needed.
Personal electronics
Although they’ve successfully been used in some applications, supercapacitors are unlikely to replace LIBs for powering small personal electronics. Even Skeleton Technologies has noted that their 3V SkelCap supercapacitors are meant to be used in combination with batteries, not as a replacement. SkelCaps have a specific energy about one order of magnitude lower than that of LIBs, meaning several would be needed to obtain the same energy density as only a single LIB. For powering small personal electronics such as smartphones and cameras, consumers are unlikely to be interested in recharging their devices after only a short duration of use, regardless of how quickly they can be recharged.
Conclusions
While they’ve found several interesting applications in the transportation sector, supercapacitors are not currently viable alternatives to lithium-ion batteries. Unless research efforts provide major breakthroughs in terms of energy density and self-discharge rate, such as by using lithium-ion hybrid supercapacitors, supercapacitors will remain complementary energy storage devices. Furthermore, any graphene-based devices are likely to experience the same pitfalls as those of non-hybrid supercapacitors due to issues surrounding graphene-active materials.
Continue exploring our resources on sustainable energy by reading “A greener future: Lithium-ion batteries and Hydrogen fuel cells.”