Gain new perspectives for faster progress directly to your inbox.
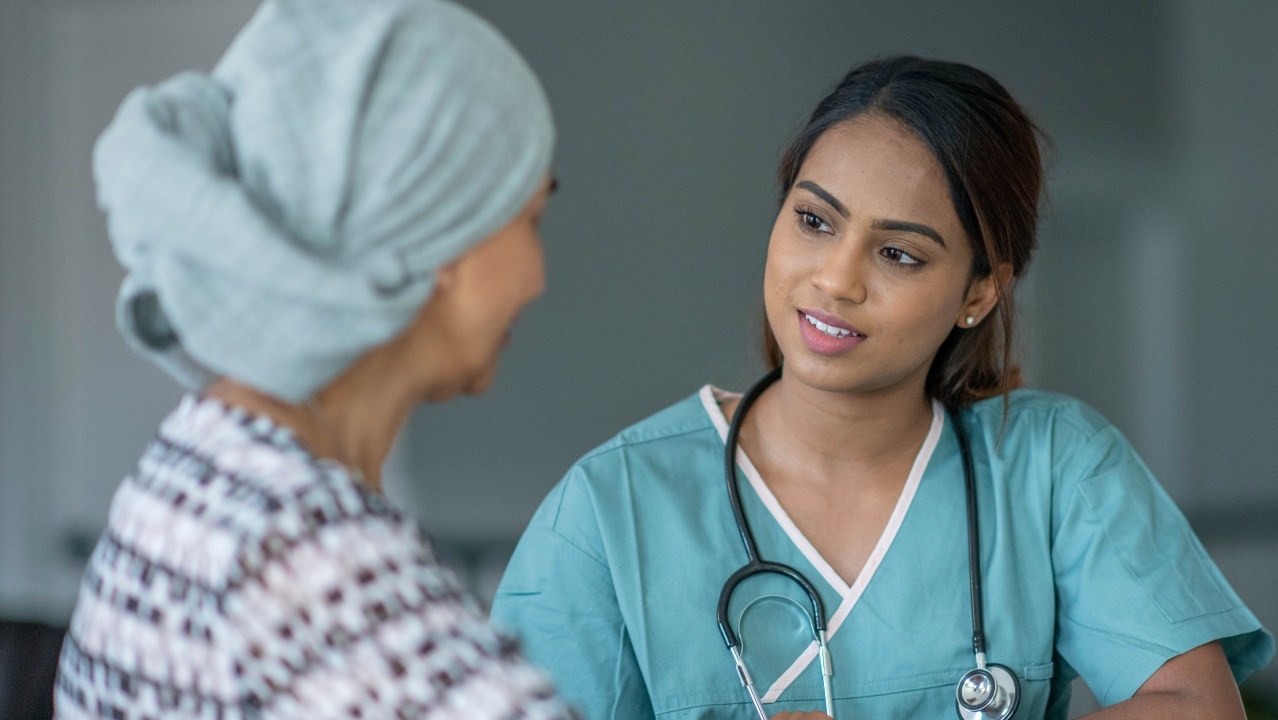
The rising global burden of cancer
The burden of cancer and associated mortality is rapidly growing worldwide, driven by an aging population as well as changes in the prevalence and distribution of the main risk factors for cancer. It is predicted that 28.4 million cancer cases will be diagnosed in 2040: a 47% rise from 2020.
Female breast cancer has surpassed lung cancer as the most diagnosed cancer, with an estimated 2.3 million new cases in 2020 (11.7%), followed by lung (11.4%), colorectal (10.0 %), prostate (7.3%), and stomach (5.6%) cancers. Immunotherapies such as checkpoint inhibitors have been a critical advancement in cancer treatment. Despite this breakthrough, immunotherapy has not been the panacea for all cancers. Not all tumor types respond to immunotherapeutic agents, and resistance mechanisms may lead to tumor immune escape and growth.
While no mRNA cancer vaccine is currently approved by the US Food and Drug Administration, breakthrough therapy designation has been granted to the investigational vaccine mRNA-4157-P201 (Moderna) in combination with checkpoint inhibitor pembrolizumab (Merck) for adjuvant therapy in high-risk melanoma following complete resection. With the success of the mRNA COVID-19 vaccines, researchers are confident that mRNA vaccine technology can be harnessed to treat cancer cells. With that said, could we be close to integrating mRNA therapy into the cancer treatment landscape?
Coming full circle — mRNA vaccines and cancer
To many, it may seem like the COVID-19 mRNA vaccines were developed overnight. Yet, the rapid design, manufacturing, and testing of these vaccines would not have been possible without years of research forming the groundwork for flu, Cytomegalovirus, and Zika vaccines.
In 1995, pivotal research demonstrated that an intramuscular injection of naked RNA encoding carcinoembryonic antigens could elicit antigen-specific antibody responses in mice. The following year, a separate study showed that mRNA-transfected dendritic cells injected into tumor-bearing mice induced T-cell immune responses and inhibited the growth of those tumors. This work paved the way for numerous studies exploring the feasibility, efficacy, and safety of mRNA-based technologies. However, until recently, instability, innate immunogenicity, and inefficient in vivo delivery have limited mRNA vaccine and therapeutic applications. A key challenge faced by researchers was how to deliver mRNA to where it needed to go; an mRNA sequence injected into the body without some form of protection would be recognized as a foreign substance and destroyed.
The rapid development of mRNA vaccines to treat the novel coronavirus, SARS-CoV-2, has helped accelerate mRNA vaccine use from bench to bedside. For instance, the Pfizer-BioNTech and Moderna vaccines demonstrated the effectiveness of utilizing lipid nanoparticles (LNPs) for delivering mRNA to target cells. At the end of 2019, stimulated by the SARS-CoV-2 epidemic, both published literature and patent applications relating to mRNA therapeutics quickly rose worldwide. After 2020, the number of published papers showed a rapid growth trend, increasing to 3,361 in 2021 and nearly 5,000 in 2022. The number of patent applications continued its upward trend after 2020, reaching 382 in 2021, and is estimated to have increased to 510 in 2022 (Figure 1).
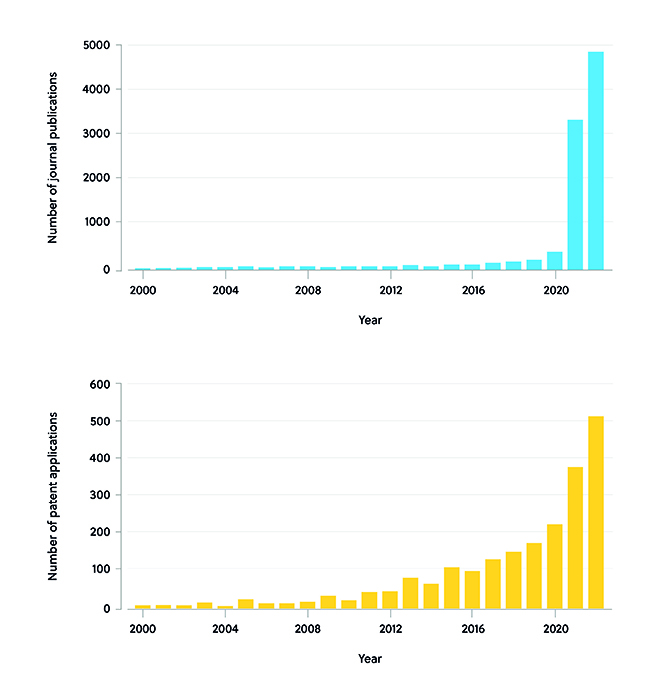
The success of the COVID-19 mRNA vaccine has revealed the potential of the mRNA platform not only for expansion to other infectious diseases but also for cancers. With insights from virus studies potentially informing work on cancer vaccines, it appears we’ve come full circle.
Recruiting the immune system — how mRNA cancer vaccines work
The applications of mRNA in cancer vaccines are broad, with researchers exploring several strategies for cancer immunotherapy:
- Antigen presentation: mRNA vaccines deliver cancer antigens to antigen-presenting cells (APCs) for the presentation of major histocompatibility complex class I and II.
- Adjuvant function: mRNA stimulates immune activation by binding to pattern recognition receptors expressed by APCs.
- Antigen receptors: mRNA introduces antigen receptors such as chimeric antigen receptors (CARs) and T-cell receptors into lymphocytes.
- Protein production: mRNA allows the expression of immunomodulatory proteins, including toll-like receptors, chemokine receptors, co-stimulatory ligands, cytokines, chemokines, and different monoclonal antibody formats into various cell subsets.
Is mRNA cancer therapy within reach?
Companies such as Genentech, CureVac, and Moderna are developing mRNA vaccines with encoding neoepitopes that can elicit immune responses against target tumors. Dozens of clinical trials are testing mRNA vaccines either as monotherapies or as part of a combination treatment in people with various types of cancer, including pancreatic cancer, colorectal cancer, and melanoma. Several candidates have entered Phase 2 trials, demonstrating favorable efficacy in melanoma, non-small cell lung cancer, and prostate cancer (Table 1).
Table 1. mRNA vaccines in cancer clinical trials (Phase 2 and beyond)
Vaccine name |
CAS Registry Number® |
Disease indication |
Antigen | Company |
Autogene cevumeran | 2365453-34-3 | Melanoma; Colorectal cancer |
Patient-specific neoantigens |
BioNTech |
mRNA 4157 | 2741858-84-2 | Melanoma | Up to 34 neoantigens | Moderna |
BNT 113 | 2882951-85-9 | PV16+ head-and-neck squamous carcinoma | HPV16-derived tumor antigens (oncoprotein E6 and E7) | BioNTech |
CV 9202 | 1665299-76-2 | Non-small cell lung cancer | NY-ESO-1, MAGE C1, MAGE C2, TPBG (5T4), survivin, MUC1 | CureVac |
CV 9103 | 2882951-83-7 | Prostate cancer | Mix of four prostate cancer-associated antigens | CureVac |
SW 1115C3 | 2882951-82-6 | Non-small cell lung cancer; esophageal cancer | Patient-specific neoantigens | Stemirna Therapeutics |
BNT 111 | 2755828-88-5 | Melanoma | Mix of four melanoma-associated antigens | BioNTech |
While mRNA cancer vaccines are garnering interest within the research community, most oncology research has historically focused on mRNA therapeutics, with a wide variety of candidates entering clinical development (Table 2), including:
- TriMix-MEL (eTheRNA Immunotherapies), a mixture of three mRNAs that activate key immune cells against cancer.
- An mRNA therapeutic (BioNTech) encoding a monoclonal antibody targeting claudin 18, a protein expressed in multiple cancers.
- An LNP-encapsulated mRNA (MedImmune LLC) administered by intratumoral injection designed to drive local interleukin-12 (IL-12) production and induce anti-tumor immunity.
Table 2. mRNA therapeutic products in cancer clinical trials
mRNA drug name | CAS Registry Number | Disease indication | Company |
TriMix-MEL; ECL-006; E011-MEL | 2877674-59-2 | Melanoma | eTheRNA Immunotherapies |
BioNTech-1; BNT 141; BNT-141; BNT141 | 2877707-22-5 | Solid tumors | BioNTech |
BNT-142; BNT142 | 2877707-34-9 | Solid tumors | BioNTech |
BNT-151; BNT151 | 2877709-82-3 | Solid tumors | BioNTech |
BNT 152; BNT152 | 2877709-92-5 | Solid tumors | BioNTech |
BNT 153; BNT153 | 2877709-93-6 | Solid tumors | BioNTech |
MEDI1191; MEDI-1191 | 2877712-03-1 | Solid tumors | Moderna |
mRNA-2752 | 2878461-50-6 | Solid tumors | Moderna |
SAR-441000 | 2879301-17-2 | Solid tumors | Sanofi, BioNTech |
SQZ-eAPC-HPV | 2879306-51-9 | HPV and solid tumors | SQZ Biotechnologies |
Making mRNA cancer vaccines a reality
We have made great strides in mRNA cancer technology in recent years, but some fundamental challenges remain. Firstly, mRNA cancer vaccines need specific packaging and delivery systems with an appropriate affinity for the target tissue/organ. Researchers are currently evaluating approaches to facilitate this, including the conjugation of organ-targeted moieties to oligonucleotides. Though LNPs are the most studied vehicles for mRNA delivery, their clinical application has been impeded by cytotoxicity concerns and their relatively short circulation time. Therefore, various alternative smart delivery systems (e.g., exosomes) are being evaluated to improve the bioavailability, loading, and release of the mRNA cargo.
Successful delivery of the mRNA cargo is not enough. To ensure maximal efficacy, researchers have been investigating approaches to enhance protein expression in vivo. All parts of the mRNA—the cap, 5′, and 3′ regions, open reading frame, and polyadenylated tail—can be optimized to augment protein expression. Chemically modified nucleosides have shown promise in this area.
In addition to the amount of protein expression, a crucial hurdle of mRNA vaccines is the relatively short period of protein production, which requires repetitive administrations. Self-amplifying and circular mRNAs are being explored as strategies to prolong the RNA lifespan and raise the total protein yield.
Though much work remains to be done, mRNA vaccines are a versatile clinical option for treating several cancer types when used alone or in combination with existing therapeutic options, such as checkpoint inhibitors. While we anticipate the arrival of the first mRNA therapeutics to market, it will be exciting to explore the outcomes from the multitude of innovative strategies seeking to tackle the global burden of cancer.
To learn more about mRNA vaccines and therapeutics, read our peer-reviewed journal publication in ACS Pharmacology and Translational Science.
Download the full Chinese report collaboration between the National Science Library of the Chinese Academy of Sciences and CAS.