Gain new perspectives for faster progress directly to your inbox.
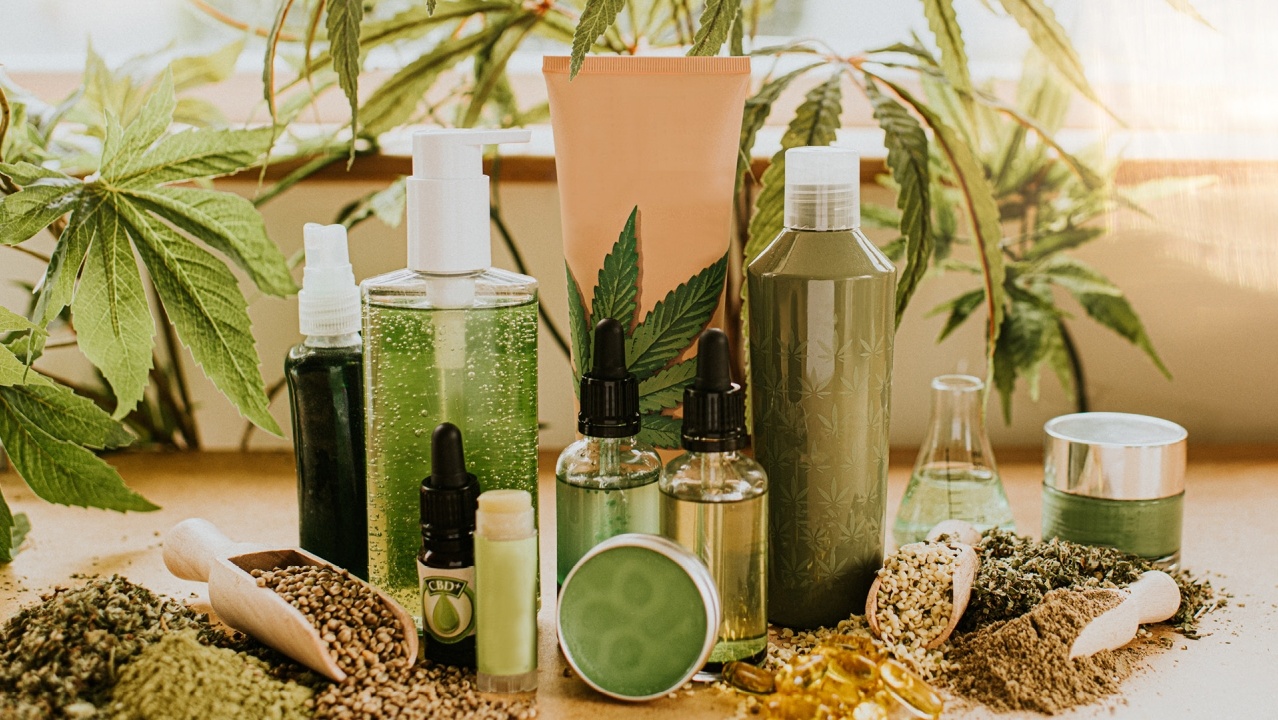
Cannabinoids are naturally occurring compounds isolated from the Cannabis sativa plant. The two best-known cannabinoids are delta-9-tetrahydrocannabinol (THC) and cannabidiol (CBD). THC is the psychoactive component of Cannabis associated with the “high.” There is an abundance of information available about THC and its derivatives, so this blog is focused on lesser-known cannabinoids, their key benefits from the scientific literature, and a deeper view into their chemical structures.
There has been a massive increase in products on the market containing CBD: oils, beauty and skin care products, therapeutic agents, beverages, chocolates, gummies, and even dog treats. This blog is not an endorsement of any of these products, and it is important to note that Cannabis is illegal at the federal level and is a schedule class I drug. However, as people are consuming these products, which are mostly marketed as “dietary supplements” and therefore no need for Food and Drug Administration (FDA) approval, it is crucial to understand their impact on human health.
Research trends in cannabinoids
Using cannabidiol (CBD) as a model cannabinoid to investigate the current research on therapeutic perspectives for cannabinoids, a quick search in CAS SciFinder yields less than 5K references.
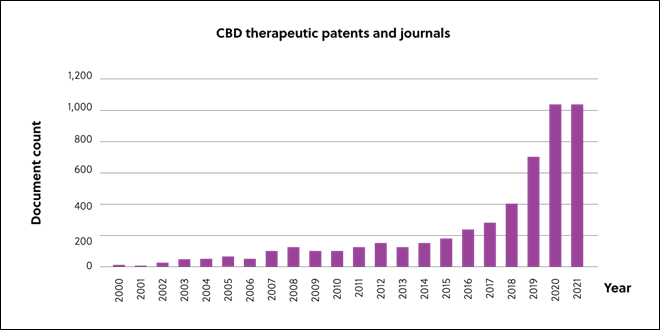
A deeper dive into the results show that human clinical studies only comprise less than 200 documents and all pre-clinical (animal, in vivo, in vitro, ADME, and in silico) studies have less than 550 results. This perhaps indicates an opportunity for drug companies, cosmetic manufacturers, nutrition organizations, and other businesses to further advance cannabinoid research for the benefit of humankind.
How do cannabinoids enter our bodies?
There are four major routes of administration:
- Inhalation
- Sublingual
- Ingestion
- Topical
One of the most popular forms of consuming cannabinoids is smoking plant material or vaping a cannabinoid oil, basically inhalation. When the cannabinoids enter the lungs, they are absorbed quickly and rapidly eliminated from the body. Inhalation tends to be a preferred method for consuming Cannabis.
Another route of administration is sublingual, where oils or tinctures containing cannabinoids are placed under the tongue are absorbed directly into the bloodstream. This method allows for faster and longer-lasting effects. Cannabinoids can also be ingested. The body will metabolize the edible forms, but this can take considerably longer to achieve the desired effects. Cannabinoids can also be used as topical agents like creams, lotions, sprays, patches, or balms. Absorption is preferred by those people who may be treating sore muscles or skin problems. Cannabinoids are absorbed through the skin directly into the bloodstream.
While THC is most widely known, a deeper understanding of the chemical structures of non-psychoactive cannabinoids like CBD, CBG, CBN, and CBC and their effects provides insight into the emerging landscape of products.
Cannabidiol (CBD)
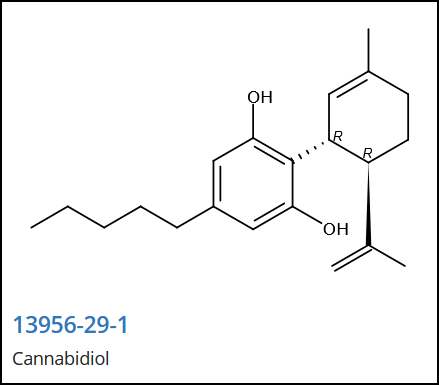
After THC, cannabidiol (CBD) is probably the most well-known cannabinoid. CBD is derived directly from the hemp plant and has no psychoactive activity. The legality of CBD is constantly in flux and each state has ever-evolving legislation regulating CBD. Harvard Medical School recognizes CBD can be used to treat anxiety, insomnia, chronic pain, arthritis, and addiction. Most importantly, CBD is a component in FDA-approved drugs to treat serious childhood epileptic diseases (ex. Epidiolex). The main side effects of CBD are nausea, fatigue, and irritability. Remember, products containing CBD are not FDA regulated and may contain impurities and unknown dosages. Exercise caution and always purchase CBD products from reputable sources.
Cannabigerol (CBG)
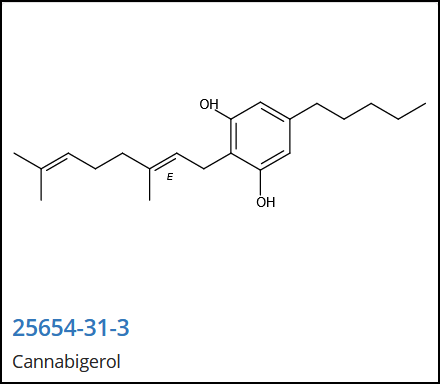
While CBG was discovered in 1964, it is used less often than CBD or THC because it is found in very low concentrations in the Cannabis plant. CBG interacts with the cannabinoid receptors in our body, namely CB1 and CB2. When the CBG attaches to these receptors, it increases neurotransmitters which affect motivation, appetite, sleep, pleasure, and pain. CBG can also affect serotonin and adrenoceptors. These receptors also control neurotransmitters—CBG is sometimes called the “bliss” molecule due to the increase in neurotransmitters. Cannabigerol has been shown to have antibiotic effects and can reduce intraocular eye pressure.
Cannabinol (CBN)
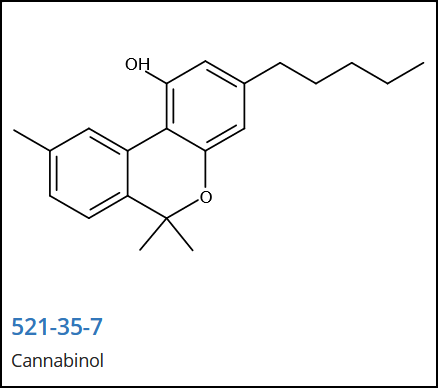
Cannabinol is not directly synthesized by the Cannabis plant; CBN is a metabolite resulting from a breakdown of THC. When the plant material is exposed to oxygen and time, CBN can increase as the THC degrades. CBN is a sedative and therefore, helps with insomnia. CBN is not well-researched, but some studies showed cannabinol to have antibiotic effects, eased glaucoma, and stimulated the appetite. In mice, CBN was shown to delay the onset of Amyotrophic Lateral Sclerosis (ALS). This promising compound offers many opportunities for researchers to pursue therapeutic uses for CBN.
Cannabichromene (CBC)
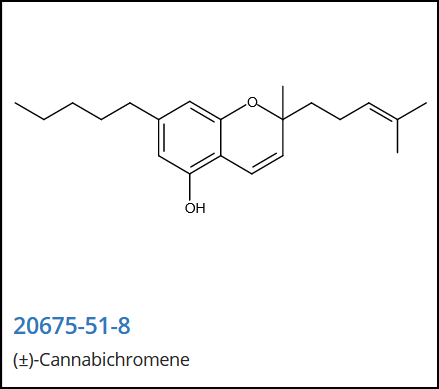
CBC is derived from CBG and has demonstrated powerful antimicrobial effects, especially in infections that have been resistant to other antibiotic treatments. In addition, some studies in rats have shown CBC to have neuroprotective effects that protect the brain from neurodegenerative conditions (Alzheimer’s Disease) and even encourage the brain to grow new cells.
CBC does not bind well with the cannabinoid receptors, but it does bind with vanilloid receptor 1 (TRPV1) and transient receptor potential ankyrin 1 (TRPA1), which are known to affect pain perception. CBC has also shown anticancer properties. Again, there is not much data on CBC as a therapeutic agent in human studies, but in preliminary research, the identified properties seem to promote further investigation.
The Entourage Effect
Many Cannabis products advertise “full-spectrum” CBD, meaning that the product not only contains CBD, but can also contain the other cannabinoids discussed here, as well as terpenes, essential oils, and up to 0.3% THC (legislated). Using these cannabinoids in conjunction with one another to increase potency and effectiveness, differing from the effects of each chemical on its own, culminates in a theory called the “Entourage Effect”. Without getting too technical, the proposed mechanism of the Entourage Effect involves inactive lipids combined with exogenous cannabinoids that increase the activity of endogenous cannabinoids (anandamide and 2-arachidonylglycerol). Research is new in this area, but some studies have shown positive results in cancer, mood and anxiety disorders, movement disorders, and epilepsy.
Future perspective and impact
Cannabinoids may have a bad rap because of their association with marijuana and the psychoactive effects of THC and its derivatives. Legal concerns may dissuade researchers from pursuing cannabinoid research, however, initial studies on cannabinoids have clear data there could be potential therapeutic benefits for these compounds, both as single components and through activating our endogenous cannabinoids and the “Entourage Effect.” This blog only addressed some of the more known cannabinoids but recall there are over 100 of these compounds identified and more to be discovered! Hopefully, with continued research, the stigma surrounding these cannabinoid substances will dissipate, and their full potential can be actualized in their treatment of debilitating diseases.
The emerging trend of increased research in recreational drugs for mainstream health benefits goes far beyond cannabinoids, see how psychedelics like LSD, Molly, and "shrooms" could be next in the fight against depression and PTSD.
Therapeutic Potential | CBD | CBG | CBN | CBC |
Antimicrobial | X | X | X | |
Anticancer | X | |||
Antianxiety | X | |||
Mood stabilizer | X | |||
Appetite stimulant | X | X | ||
Antiseizure | X | |||
Movement disorder | X | |||
Pain | X | X | X | |
Sedative | X | |||
Insomnia | X | X | ||
Neuroprotectant | X | |||
Arthritis | X | |||
Addiction | X | |||
Glaucoma | X | X |